The Sudbury Neutrino Observatory (SNO), a 1000 ton heavy water
Cherenkov detector under
construction in INCO's Creighton mine near Sudbury, Ontario (Canada)
Neutrino detectors
Neutrino interactions
with matter are extremely
rare, making detection difficult.
Neutrino detectors are typically large tanks filled with a
fluid that reacts to the passage of neutrinos.
To take advantage
of the high flux of neutrinos passing through the Earth
(billions per second),
neutrino detectors are made as large as possible. The
larger the detector, the more neutrinos can be
measured in a reasonable amount of time.
For example the
Super-Kamionkande
device under construction in Japan is designed to detect about
11,000 neutrinos per year.
Early neutrino detectors were filled with perchloroethane
(a type of cleaning fluid containing chlorine).
A portion of the chlorine is isotope 37
(17 protons and 20 neutrons), which
can react with neutrinos to produce
Argon-37 (18 protons and 19 neutrons).
The amount of Argon-37 created is then used to
measure the neutrino flux.
Another fluid used for neutrino detection is water.
Neutrino interactions in water produce the
Cherenkov effect.
Light emitted in this manner is detected
by sensitive detectors surrounding the fluid.
Cosmic rays can cause unwanted signals in these detectors.
To avoid this problem, the detectors are put deep
underground, so that layers of rock can shield
the detector from cosmic rays.
You might also be interested in:

Super-Kamiokande is a water Cerenkov detector located in the Kamioka Mozumi mine in Japan. Its use includes proton decay studies, neutrino detection (from the Sun, the atmosphere and supernovae) and muon
...more
The theory of relativity states that no particle can travel at the speed of light in a vacuum. However, light travels at lower speeds in dense media, like water. A particle traveling in water must have
...more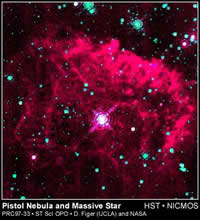
Fusion in the core of the stars is achieved when the density and temperature arising from the gravitational pressure are high enough. There are different fusion cycles that occur in different phases of
...more
The Sun, as well as other stars, releases energy in the form of radiation and particles. The processes that produce this energy are taking place in the interior of the Sun, where direct observations are
...more
With just our eyes, we can see many things in the night sky, including stars, planets, meteors, comets, auroras, and the Moon. Have you ever looked up and wished that you could take a closer look at the
...more
Neutrino interactions with matter are extremely rare, making detection difficult. Neutrino detectors are typically large tanks filled with a fluid that reacts to the passage of neutrinos. To take advantage
...more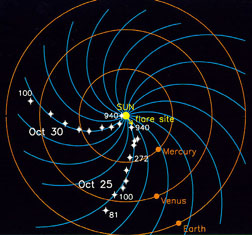
IMF stands for Interplanetary Magnetic Field. It is another name for the Sun's magnetic field. The Sun's magnetic field is enormous and is carried by the solar wind. The solar wind and magnetic field are
...more
The basic Hydrogen fusion cycle involves four Hydrogen nuclei (protons) and two electrons and yields a Helium nucleus, two neutrinos and six photons. This process occurs in three steps: the first one is
...more